Understanding Battery Technologies in Electric Vehicles
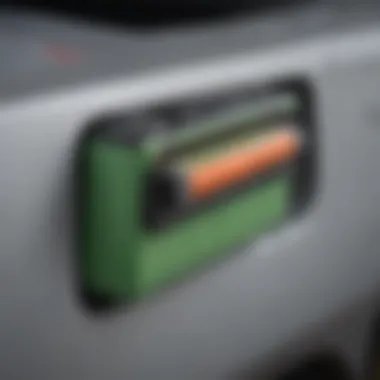
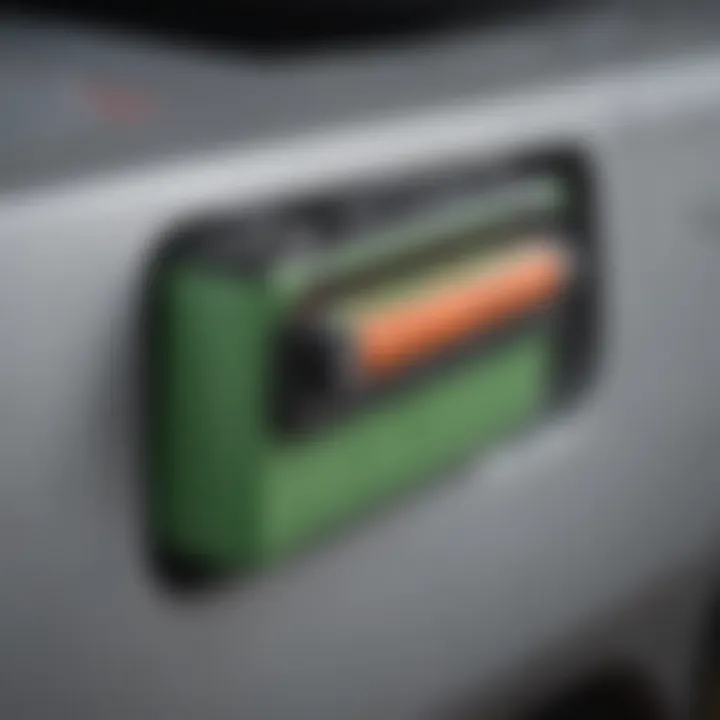
Intro
The automotive world is changing. Electric vehicles (EVs) are gaining attention and reshaping the market. At the center of this shift are the batteries that power these vehicles. Different battery types bring unique features, strength, and challenges. Understanding these can be essential for consumers and enthusiasts alike. From sedan lovers to eco-conscious buyers, various individuals benefit from knowing battery systems.
Batteries are important for energy efficiency, sustainability, performance, and safety. This article navigates through different battery types. Key focuses will be lithium-ion, solid-state solutions, and potential alternative options. Such exploration allows for informed decisions when it comes to EV ownership.
Auto buyers now face a plethora of choices. The familiar gas-powered car must be weighed against electric alternatives. The right information can ensure a choice that balances cost, features, and environmental impact. Knowledge of battery technologies is pivotal in this evolving sector.
Understanding the nuances will range beyond just the physical components themselves. Future of automotive mobility is closely linked with charge times, range per charge, and the ecological aspects of common batteries. Let's begin with a deeper look at the specific vehicle reviews based on these battery technologies.
In-Depth Vehicle Reviews
Overview of the Vehicle
The number of electric vehicles is increasing. Models like Tesla Model S, Nissan Leaf, and Chevrolet Bolt are already common sights on roads. Each utilizes different battery setups, affecting how they operate. Tesla relies on a complex lithium-ion setup to ensure maximum range. On the other hand, Nissanβs Leaf keeps its design simple yet effective, focusing more on accessibility and everyday commuting.
Key Specifications
Key specifications of these EVs show important insights. A selection of features often highlights battery capacity, charging speed, and range.
- Tesla Model S: 100 kWh battery, over 370 miles of range, quick charge capability.
- Nissan Leaf: 40 kWh battery; about 150 miles of range; focuses on daily driversβ needs.
- Chevrolet Bolt: 66 kWh battery; around 259 miles per charge, designed for efficiency and driver experience.
Each carβs battery integrates into its whole design, impacting power delivery and environmental consideration.
Performance Analysis
Performance is central to any car choice. Tesla's powerful lithium-ion battery systems offer vigorous acceleration and distance coverage. In terms of electric range, Tesla generally outperforms its rivals, especially during long-distance trips.
Meanwhile, the Nissan Leaf, designed for urban users, offers moderate acceleration yet satisfies most city driving. The Chevrolet Boltβs robust performance often leads to a well-rounded experience without compromising battery life or safety.
Safety Features
Battery safety cannot be overlooked. With developing technologies, manufacturers prioritize designs to mitigate risks.
- Tesla models come fortified with thermal management that helps prevent overheating.
- Nissan Leaf is equipped with an array of sensor systems for collision avoidance and stability control.
- Chevrolet Bolt incorporates rugged casing against impact, ensuring structural safety.
Technology and Infotainment
Modern EVs go beyond propulsion. Each brand enhances user experience through technology integrations, featuring infotainment systems and navigation tools.
- Teslaβs interface retains top status, providing a user-friendly layout with continual software updates.
- Nissan stays functional, integrating technology without excess complexity.
- Chevrolet players bingo on in-car options enhancing driving enjoyment without distractions.
This technological push affects battery optimization and overall vehicle usability.
Fuel Efficiency and Sustainability
When it comes to fuel use, EVs more smartly transform electrical energy into motor capability. Findings suggest cost per dimensional unit materialize in lower fossil oil demands.
Keeping a clear focus on longevity, adopting environmentally friendly sourcing materials is effective. Recent efforts are aimed at minimizing waste and increasing regenerability on battery production, bolstering automatic economy nationally.
Understanding battery technologies and their impacts improves informed decision-making for potential buyers before anything else.
Further discussing specifics around these vehicles unlocks clarity and enriches discussions regarding their roles in energy evolution.
Foreword to Electric Vehicle Batteries
Electric vehicles (EVs) are fundamentally changing the automotive landscape, with battery systems at the core of these advancements. Understanding electric vehicle batteries is crucial for both consumers and industry professionals. It involves recognizing how the batteries operate, their types, and the significant role they play. With various battery technologies underpinning todayβs EV models, knowledge in this area is vital for informed choices and technological advancements.
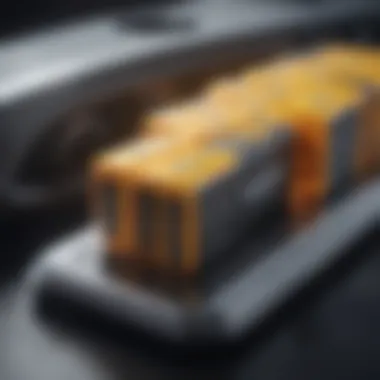
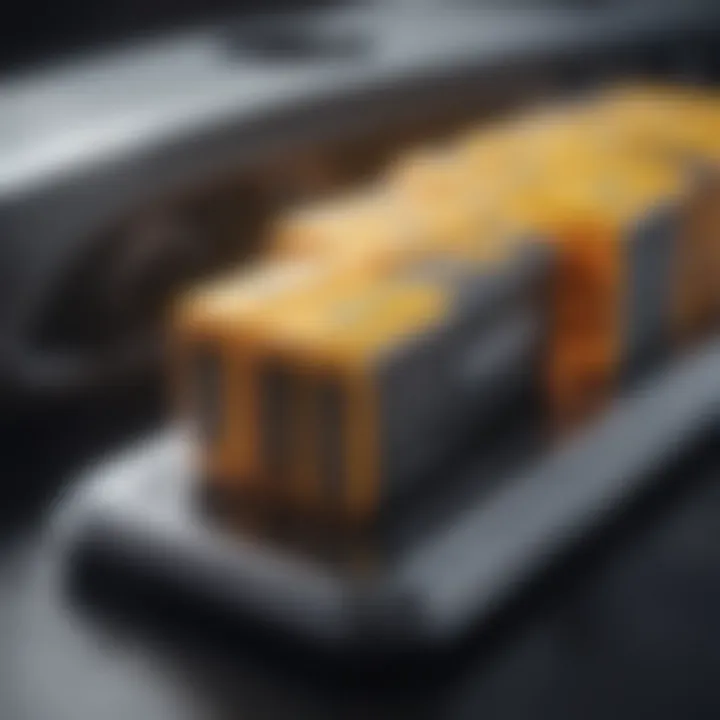
The Role of Batteries in Electric Vehicles
Batteries serve as the lifeblood of electric vehicles, powering the electric motors that drive the wheels. Their function transcends mere energy provision; they also influence vehicle performance, range, charging speed, and overall efficiency. For example, how long an EV can travel on a single charge depends significantly on the battery technology used. Battery efficiency in converting stored energy into power can determine an EV's acceleration capability as well.
Additionally, batteries also affect safety standards and vehicle weight. These factors become increasingly important as designers seek to align EV performance with traditional cars.
βThe battery technology determines the driving dynamics and long-term viability of electric vehicles in the evolving automotive market.β
Furthermore, as advancements in technology enable deeper integration with other vehicle systems, the role of batteries is expanding even further.
Overview of Battery Technology Evolution
The evolution of battery technology is marked by significant milestones. Initially, lead-acid batteries were common, known for their simplicity and affordability. However, they limit energy density and efficiency, making them less suitable for todayβs needs. Over time, the progression to nickel-metal hydride and then lithium-ion technologies revolutionized the industry.
- Lithium-ion batteries, the current standard, offer higher energy densities and cycling stability compared to earlier technologies.
- Moreover, research is aimed at improving these existing systems and developing alternatives such as solid-state batteries, which may outpace current options in performance.
Emerging technologies are positioned to impact the future of electric vehicle batteries significantly. Innovations are driving not just hardware improvements but also software management systems that ensure optimum performance, longevity, and sustainability. These changes correlate with a demand for EVs to become mainstream, emphasizing the ongoing cultivation of efficient battery systems and other technologies.
Lithium-Ion Batteries: The Current Standard
Lithium-ion batteries are often seen as the cornerstone of electric vehicle technology today. Their ability to store and deliver significant amounts of energy makes them a dominant choice in the design of electric vehicles. This section delves comprehensively into their composition, advantages, and the various challenges that they face.
Composition and Structure
Lithium-ion batteries consist of several key components that allow them to perform efficiently. The fundamental structure includes two electrodes, the anode and the cathode. The anode is usually made from graphite, while the cathode often consists of lithium metal oxide materials. The electrolyte, typically a lithium salt in an organic solvent, facilitates the movement of lithium ions between the two electrodes during charge and discharge cycles.
The performance and longevity of a lithium-ion battery can be substantially influenced by these materials. Various formulations exist; for instance, lithium nickel cobalt aluminum oxide (NCA) and lithium nickel manganese cobalt oxide (NMC) are common cathode materials each selected based on specific performance metrics. This careful selection of component makeup is vital to meet the demanding requirements of modern electric vehicles.
Advantages of Lithium-Ion Batteries
Lithium-ion batteries offer numerous benefits that contribute to their prominence in the automotive industry:
- High Energy Density: They can store a considerable amount of energy relative to their weight, enhancing the driving range of electric vehicles.
- Efficiency: With low self-discharge rates and high charge/discharge efficiency, lithium-ion batteries maintain energy levels effectively.
- Durability: They can endure many charge cycles without significant degradation, making them suitable for long-term usage in electric vehicles.
- Rapid Charge Capability: Many lithium-ion batteries support fast charging, significantly reducing downtime for drivers.
These features make lithium-ion batteries appealing not just for electric cars but also for various everyday electronics, establishing their dominance.
Challenges and Limitations
Despite their advantages, lithium-ion batteries also face several limitations that are worth noting:
- Resource Dependence: The mining of lithium, cobalt, and other materials raises environmental and ethical concerns.
- Temperature Sensitivity: Extreme temperatures can affect battery performance and longevity, posing challenges for certain climates.
- Fire Risk: Lithium-ion batteries are prone to thermal runaway, which can lead to fire hazards if not properly managed.
- Recycling Issues: Although improving, recycling lithium-ion batteries efficiently remains a technical challenge. This can create waste management issues.
These challenges underline the need for innovation in battery technology. Advancements in various alternatives continue to focus on improving sustainability, safety, and overall world environmental impacts.
Solid-State Batteries: The Next Frontier
Solid-state batteries represent a pivotal advancement in battery technology, especially in the context of electric vehicles. As the automotive industry strives towards greater efficiency, safety, and sustainability, solid-state technology emerges as a crucial player. With a transition from traditional lithium-ion setups to this new technology, it's essential to understand the principles, advantages, and existing challenges related to solid-state batteries.
Basic Principles of Solid-State Technology
Solid-state batteries utilize solid electrolytes instead of liquid ones. Traditional lithium-ion batteries typically have a liquid or gel electrolyte that enables lithium ions to move between the anode and the cathode. In contrast, solid-state batteries implement solid materials in the same role. This shift leads to several potential benefits, which are increasingly important contrasted with conventional batteries.
The solid electrolyte can be made from various materials, including ceramics and glass. These materials allow for a denser packing of energy per volume, offering higher energy densities. This is one reason manufacturers view solid-state technologies as the future of energy storage. The elimination of liquid mediums also reduces the risks associated with leaks and battery swelling, adding a layer of safety for the end-user.
Potential Benefits Over Lithium-Ion Batteries
Several characteristics of solid-state batteries position them favorably against their lithium-ion counterparts:
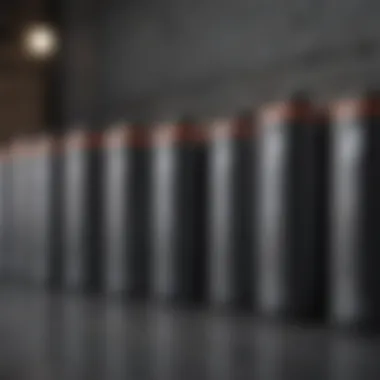
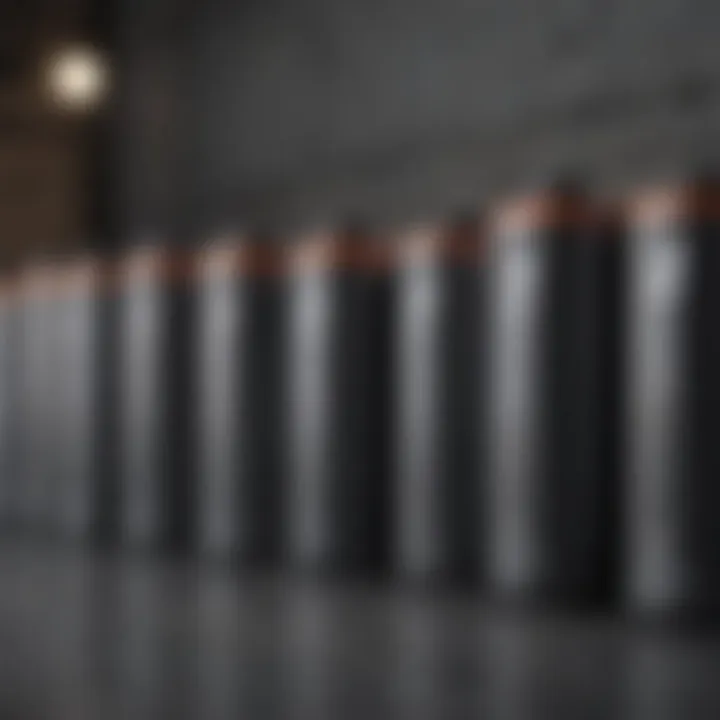
- Higher Energy Density: The capacity for energy storage is superior in solid-state batteries. This leads to longer driving ranges for electric vehicles on fewer charges.
- Improved Safety: The use of solid electrolytes reduces flammability issues seen with liquid electrolytes. Fewer fire hazards can enhance consumer confidence in EVs.
- Better Longevity: Solid-state batteries can endure more charging cycles with less degradation. This leads to longer battery life, which is vital for both manufacturers and consumers.
- Faster Charging: The ionic movement in solid systems can potentially increase charging speed, an important aspect for user convenience.
Expectations for the arrival of commercial solid-state batteries include a shift towards leaner vehicle designs and enhanced performance that aligns with growing environmental regulations.
Current Development Challenges
Despite their promise, solid-state batteries face significant hurdles and technical challenges that need addressing before mass production is feasible. Some of the critical issues include:
- Manufacturing Complexity: The production of solid-state batteries is challenging and expensive. Developing scalable manufacturing processes is essential to reduce costs.
- Material Limitations: The selection of suitable materials that can function at various temperatures is still ongoing. Ensuring materials support long-term performance without issues remains a research focus.
- Interfacial Resistance: Solid-state batteries often encounter challenges with voltage over time, caused by the interface between the solid electrolyte and electrodes. Managing this gap is crucial for performance.
- Early-Stage Adoption: Solid-state technology is still in its infancy, leading to questions about reliability and overall widespread use. Initial reluctance among manufacturers to switch could keep production lower than required to penetrate the market.
Overall, solid-state batteries mark a decisive turn in battery technology. Transitioning from traditional lithium-ion systems to this innovative architecture holds great promise. However, without resolving existing hurdles, the awaited advances might not materialize as expected. Understanding these aspects is vital for those involved in electric vehicle technology and innovation.
Alternative Battery Technologies
Alternative battery technologies are gaining traction as the electric vehicle market evolves. These technologies offer additional options to the current dominant lithium-ion batteries. Crucially, they promise to meet various user needs effectively while focusing on safety, sustainability, and cost-efficiency. Exploring these alternatives not only highlights their unique benefits but also point to the future direction of automotive technology.
Lithium Iron Phosphate (LFP)
Lithium Iron Phosphate, often shortened to LFP, presents an interesting alternative to traditional lithium-ion batteries. This type of battery utilizes iron as its primary cathode material, making it distinct from other battery types.
One major advantage of LFP batteries is their outstanding thermal stability. They do not overheat easily and offer enhanced safety, which makes them a useful choice in vehicle applications. Additionally, LFP batteries tend to have a significantly longer lifecycle, often exceeding thousands of charging cycles before losing a substantial portion of their capacity. This leads to longevity in service, which can be economically beneficial for electric vehicle owners.
However, there are also challenges. LFP batteries usually have a lower energy density compared to their lithium-ion counterparts, which means they may offer relatively shorter ranges for electric vehicles. Yet, with the right applications, their benefits can outweigh these downsides.
Nickel Cobalt Manganese (NCM) Batteries
Nickel Cobalt Manganese, often referred to as NCM batteries, is another popular alternative in the realm of electric vehicle batteries. This technology integrates nickel, cobalt, and manganese in its composition, striking a balance between cost, performance, and lifecycle.
NCM batteries are exceptionally energy-dense and can offer performance similarities or even superiority to traditional lithium-ion batteries. Increased energy density enables EV manufacturers to design vehicles with longer ranges without considerably increasing weight. Additionally, these batteries can be customized for specific performance needs by adjusting the ratios of nickel, cobalt, and manganese.
However, the reliance on cobalt raises an ethical concern. The mining process can often have negative societal and environmental impacts. As such, some manufacturers are exploring ways to minimize cobalt content or find similar performance materials through innovative research and development.
Emerging Technologies in Battery Design
The race in the electric vehicle market prompts consistent development of emerging battery technologies. Innovations like lithium-sulfur, aluminum-air, and flow batteries show promise as future alternatives that could redefine performance standards.
Lithium-sulfur batteries could potentially provide even greater energy density than traditional lithium-ion options. Theoretically, they may reach almost double the capacity, leading to longer driving ranges once fully perfected. Aluminum-air batteries, while less common in mobile applications, offer efficient energy when needed, deriving a large portion of their energy from aluminum oxidation. Flow batteries, mainly advantageous in larger scale applications, allow energy to be stored in external tanks and redirected when necessary, differing significantly from conventional battery setups.
Emerging technologies focus not only on performance but also on environmental concerns. Researchers continually aim to use abundant materials, generate less waste, and contribute to a circular economy in electronics.
Battery Management Systems (BMS)
Battery Management Systems (BMS) play a critical role in the effective functioning of electric vehicles. They not only manage the battery cells but also ensure the safety, performance, and longevity of electric vehicle batteries. Without a robust BMS, electric cars would struggle in maintaining their efficiency and reliability. This systems are therefore central to understanding electric vehicle technology.
Functions and Importance of BMS in EVs
BMS serve several important functions crucial for the operation of electric vehicles. Their primary role is to monitor the battery's status and prevent malfunction.
- Cell Monitoring: BMS continuously checks the voltage and temperature of each individual cell within the battery pack. It prevents any cell from overcharging or overheating, thus ensuring high safety standards.
- State of Charge (SOC) Estimation: The BMS is capable of calculating the current charge level of batteries. Proper knowledge of SOC helps in making informed decisions regarding charging and usage.
- Thermal Management: Proper thermal management is important for long battery life. BMS helps maintaining ideal temperature settings, which ensures performance and longevity of battery cells.
- Balancing: During operation, battery cells can become unbalanced. BMS routinely performs cell balancing to make sure all cells share the same capacity, enhancing the battery's overall performance.
The significance of a BMS extends beyond performance metrics. They also influence safety measures. By preventing dangerous situations like thermal runaway or fire hazards, BMS can be seen as the protector of battery integrity.
Integration with Electric Drive Systems
The integration of BMS with electric drive systems is essential for optimized vehicular control. A talented BMS interfaces seamlessly with control strategies used in electric drive units. This integration results in improved operational efficiency and safety for the electric vehicle.
With the electric drive system's data, BMS informs on the power requirements. It adjusts the output to achieve optimal efficiency. The mutual interaction enhances all aspects of energy consumption - battery life, driving range, and overall performance.
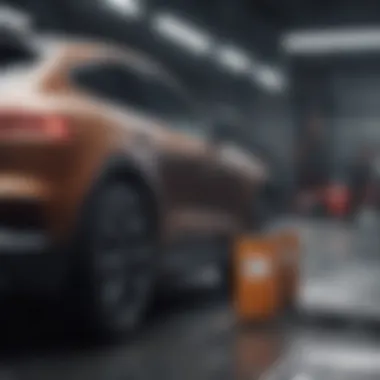
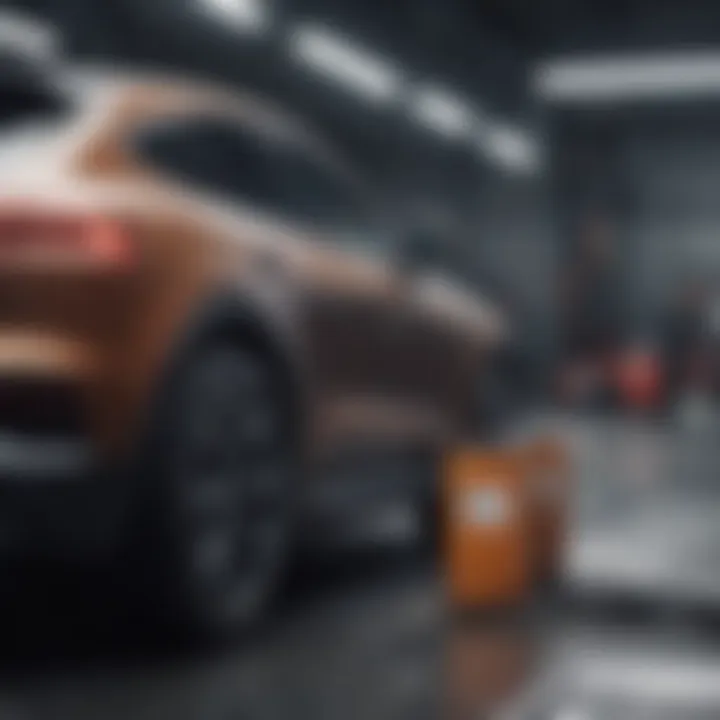
Effective BMS drives advancements in electric vehicle capabilities and shapes the future of automotive technology.
Environmental Considerations
The topic of environmental considerations around electric vehicle batteries is profoundly significant. As the automotive industry evolves, acknowledging the environmental impact is crucial for sustainable progress. Cars powered by electricity have the potential to reduce emissions. However, their battery production and eventual disposal also carry ecological consequences.
Key elements include understanding the battery lifecycle, from mining raw materials to potential recycling methods. As vehicles gain popularity, assessing their overall environmental impact becomes essential for consumers and manufacturers alike.
Lifecycle Analysis of EV Batteries
Lifecycle analysis refers to evaluating the impacts of an electric vehicle battery throughout its existence. This process typically includes several phases: raw material extraction, manufacturing, use, and end-of-life management. Each phase holds challenges and dimensions important to understanding the entire picture.
- Raw Material Extraction: Battery production requires raw minerals, such as lithium, cobalt, and nickel. Mining these materials can result in habitat destruction, pollution, and excessive water consumption. The environmental consequences of these practices must be scrutinized.
- Manufacturing: The battery manufacturing process consumes significant energy, often derived from fossil fuel sources. This phase can produce greenhouse gas emissions, thereby impacting climate change.
- Use Phase: During the actual use of electric vehicles, emissions drop, especially compared to gasoline-powered cars. A cleaner energy mix contributes positively during this phase. Yet, there are regional differences that might influence results.
- End-of-Life Management: Finally, considering what happens during disposal or repurposing of depleted batteries is vital. Without proper channels for recycling, harmful materials might leach into the environment.
Recycling and Sustainability Efforts
Recycling and sustainability efforts for electric vehicle batteries play a critical lole in mitigating their environmental impact. As the usage of electric vehicles soars, finding efficient recycling methods becomes paramount.
Some of the key elements include:
- Resource Preservation: Effective recycling can recover valuable materials like lithium and cobalt, reducing reliance on new raw material sources.
- Reduced Pollution: When batteries are properly recycled, harmful chemical potential risks to ecosystems decrease significantly.
- Second-Life Applications: Used batteries can serve secondary purposes post their vehicle operational phase. For instance, they can be utilized in energy storage systems for homes.
- Innovative Technologies: Advances in recycling methodologies arise steadily. Companies are working on more efficient processes to recover battery materials and renew their life-cycle.
In a broader sense, ongoing research and developments in battery recycling can pave the way for an eco-friendlier automotive industry. Consumers and manufacturers need to emphasize and adopt sustainable practices. As part of this growing dialogue, these initiatives can profoundly alter the environmental impact of electric vehicle batteries.
The future of electric vehicle batteries sits at the intersection of technological advancement and environmental stewardship. Sustainability should lead the direction for development across sectors.
Future Trends in Battery Technology
The advancement of battery technologies is vital for the continued growth of the electric vehicle (EV) industry. Future trends in this space promise not only to improve performance but also to impact various sectors of society. Key components include efficiency, sustainability, and technological evolution that drive innovation in the automotive sector.
Predicted Advances in Battery Performance
The future of battery performance appears promising. Among the areas of development, energy density stands out. Higher energy density means cars can travel further on a single charge. Fast charging technologies are also likely to improve. This means an EV could add significant range in a short duration, which would appeal to many drivers.
Lithium-sulfur and solid-state batteries are expected to emerge as strong contenders. These technologies have unique characteristics, including greater ability to store energy relative to their weight. Further, peak performance under various weather conditions can separate leading battery technologies from the rest of the field.
Advancements may not only focus on performance but also drop costs. Battery replacements can be one of the significant expenses for EV owners. In the coming years, manufacturers like Tesla and BYD are expected to pursue innovative manufacturing techniques that can lower production costs. These improvements may directly reflect in the retail price of electric vehicles.
Impact of Regulatory Changes on Battery Development
Regulatory frameworks will shape the future landscape of battery technology significantly. Governments worldwide are currently enacting stricter emissions regulations and incentives for EV adoption. These developments drive manufacturers to invest in advanced battery solutions.
Regulations on battery disposal and recycling are also gaining attention. Governments are focusing on sustainability, which leads to advancements in recycling technologies. Enhanced regulations will ensure that manufacturers are accountable for the longevity and fate of their products.
Also, different countries have varying approaches to regulation, affecting battery technology development in complex ways. For instance, the European Union emphasizes recycling targets, setting a benchmark for sustainability that US policy may eventually follow.
On the consumer level, regulatory incentives may motivate buyers to consider EVs over traditional combustion-engine cars. Tighter mandates on fuel efficiency can help this shift. This push affects not only demand for EVs but also accelerates research and innovation in broader battery technology.
The End
In today's automotive landscape, understanding the batteries powering electric vehicles is crucial. This discussion has explored multiple battery types, revealing not just their diverse characteristics but also their roles in shaping the future of transportation.
Recap of Current and Future Battery Technologies
We delved into lithium-ion batteries as the current standard, and highlighted the benefits they offer, such as efficiency, energy density, and relative safety. Additionally, solid-state batteries were presented as a promising alternative, characterized by their higher energy density and improved safety measures. Emerging technologies like lithium iron phosphate and nickel cobalt manganese were discussed, each offering unique advantages that cater to different market needs.
Moreover, advances in battery technology will likely necessitate a regulatory response, which will further shape innovation and adoption in the market. Monitoring these changing trends allows stakeholders to stay informed and relevant in a rapidly evolving sector.
Significance for Automotive Innovation
The battery type installed in an electric vehicle directly influences its performance and sustainability. As manufacturers seek to enhance vehicle range and reduce charging times, investing in batter technology is essential. Innovations do not just aim for technical improvement but also consider environmental impacts. New materials and design standards play a significant role in enhancing sustainability in vehicle manufacturing.
The ability to handle energy fluctuations efficiently through smart energy management systems ensures that electric vehicles can operate effectively without compromising user experience. Todayβs auto innovation isn't only about how fast or long a vehicle can move; it is about how responsibly we can do it. As companies prioritize research in battery science, they shift towards a more integrated approach to climate responsibility and operational excellence.
"Understanding how the technologies function is vital not just for manufacturers but also consumers gauging the sustainability and efficiency of their transportation choices." This insight into battery types forms a core part of grasping contemporary developments in the EV market, creating informed participants in the new automotive era.